Contact
321 Reed McDonald Building
Ph: 979-845-7048
Mailing Address:
Department of Chemistry
Texas A&M University
P.O. Box 30012
College Station, TX 77843-3012
Shipping Address:
Department of Chemistry
Texas A&M University
580 Ross Street
MS3255 TAMU
College Station, TX 77843-3255
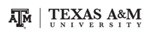
Research
Current Projects -- Press Clippings -- Videos -- Covers -- Safety
Current Projects
Research in the Gladysz group has traditionally been centered
around organometallic chemistry, and from this core area branches into
catalysis, organic synthesis, enantioselective reactions, stereochemistry,
mechanism, and materials chemistry. Currently, about half of the group is
involved with catalysis projects, divided 50:50 between structurally novel
enantioselective catalysts and highly fluorinated recoverable catalyst systems.
The other half designs organometallic building blocks for the synthesis of
molecular wires, compasses, and gyroscopes.
One active area involves complexes in which wire-like sp-carbon chains span
two transition metals. There is the obvious question of how long a polyalkyne
or (C≡C)n bridge can be synthesized. As shown in Scheme 1, PtCxPt complexes
with as many as twenty-eight carbons are easily isolated – shattering the
record for polyynes previously held by carbon or silicon endgroups.1
Scheme 1. Synthesis of Diplatinum Polyynediyl Complexes with Even Numbers of C≡C Bonds: (a) wet n-Bu4N+ F-/Acetone; (b) ClSiMe3; (c) cat. CuCl/TMEDA, O2, Acetone.
Because of the metal termini, all such species are redox-active. This in turns raises the possibility of a variety of charge-transfer phenomena involving the metal termini. In order to help stabilize certain charged redox states, we have sought to "insulate" the sp-carbon chains. The two approaches in Scheme 2 yield novel double-helical diplatinum complexes of the type I, in which sp3-carbon chains coil around the sp carbon chain.2 Such compounds are now being tested as "molecular wires". Figure 1 illustrates the impressive degree of steric protection.
Scheme 2. Syntheses of Double-Helical Diplatinum Complexes
Figure 1. Space Filling Representation of a Typical Double-Helical Diplatinum Complex
In Scheme 2, it was a pleasant surprise that alkene metathesis could be applied within a metal coordination sphere. This led to the consideration of other possible uses. For example, gyroscopes have numerous technological applications, but until our work no molecules that mimic the symmetry, connectivity, and rotational abilities of common toy gyroscopes were known. In a synthetic tour-de-force, a three-fold alkene metathesis reaction was used as shown in Scheme 3 to access families of complexes with the structures III and IV.3 Views of the crystal structure of III are supplied in Figure 2.
Scheme 3. Syntheses of gyroscope-like molecules.
Figure 2. ORTEP (top) and space-filling (bottom) representations of the crystal structure of III (n = 6).
NMR measurements show that the Fe(CO)2(NO) moiety in IV rotates within the methylene cage at an effective rate of 1000000 rpm at room temperature. This work has been extended to other metals and rotating groups, as well as much larger cage sizes. Some recent results with a similarly-synthesized platinum gyroscope are illustrated in Scheme 4. In collaborations with physicists, possible nanotechnological applications are being probed.
Scheme 4. Reactions of a Platinum-Containing Gyroscope-like Molecule
In enantioselective catalysis, ferrocene-containing chiral ligands play a major role. We have sought to incorporate a wider range of "spectator" metal fragments that feature unique architectural and electronic properties into donor ligands. Typical examples of ligands and/or their complexes are shown in Figure 3.4 These have provided exceptionally effective rhodium and palladium catalysts for enantioselective hydrogenations, hydrosilylations, and related processes. Furthermore, the rhenium-containing phosphine VII has recently been shown to be an effective "organocatalyst" for various transformations, two of which are depicted in Scheme 5.
Figure 3. Ligands that contain a chiral rhenium fragment, and/or complexes there of.
Scheme 5. Enantioselective Catalysis using Chiral, Rhenium-Containing Phosphines
Another area of current interest involves "fluorous" chemistry. When sufficient numbers of "ponytails" (CH2)mRfn (Rfn = (CF2)n-1CF3) are added to catalysts or reagents, they acquire exceptional affinities for perfluoroalkanes and other fluorous phases. Furthermore, they also commonly exhibit highly temperature-dependent solubilities in organic solvents. As shown in Scheme 6, reactions can often be conducted under homogeneous conditions at elevated temperatures, and the catalyst recovered by a liquid/solid phase separation at lower temperature.
Scheme 6. Recovery of Fluorous Catalysts via Solid/Liquid Phase Separation.
For laboratory-scale reactions involving efficient catalysts, it is often only necessary to recover a few milligrams of material. To facilitate such separations, fluorous supports have been investigated. Of these, the most interesting proves to be common laboratory Teflon tape. In fact, the catalyst can even be precoated on the tape, allowing delivery to be controlled by length. As shown in Scheme 7, a rhodium complex containing fluorous phosphines (IX) desorbs at 55 °C in dibutyl ether, catalyzes the hydrosilylation of ketones, and reprecipitates onto the tape upon cooling.5
Scheme 7. Recycling of a Thermomorphic Fluorous Rhodium Hydrosilylation Catalyst using Teflon® Tape.
(1) Zheng, Q.; Gladysz, J. A. J. Am. Chem. Soc. 2005, 127, 10508.
(2) Stahl, J.; Bohling, J. C.; Bauer, E. B.; Peters, T. B.; Mohr, W.; Martín-Alvarez, J. M.; Hampel, F.; Gladysz, J. A. Angew. Chem., Int. Ed. 2002, 41, 1871; Angew. Chem. 2002, 114, 1951.
(3) Shima, T.; Hampel, F.; Gladysz, J. A. Angew. Chem., Int. Ed. 2004, 43, 5537; Angew. Chem. 2004, 116, 5653.
(4) Friedlein, F. K.; Hampel, F.; Gladysz, J. A. Organometallics 2005, 4013.
(5) Dinh, L. V.; Gladysz, J. A. Angew. Chem., Int. Ed. 2005, 44, 4095; Angew. Chem. 2005, 117, 4164.